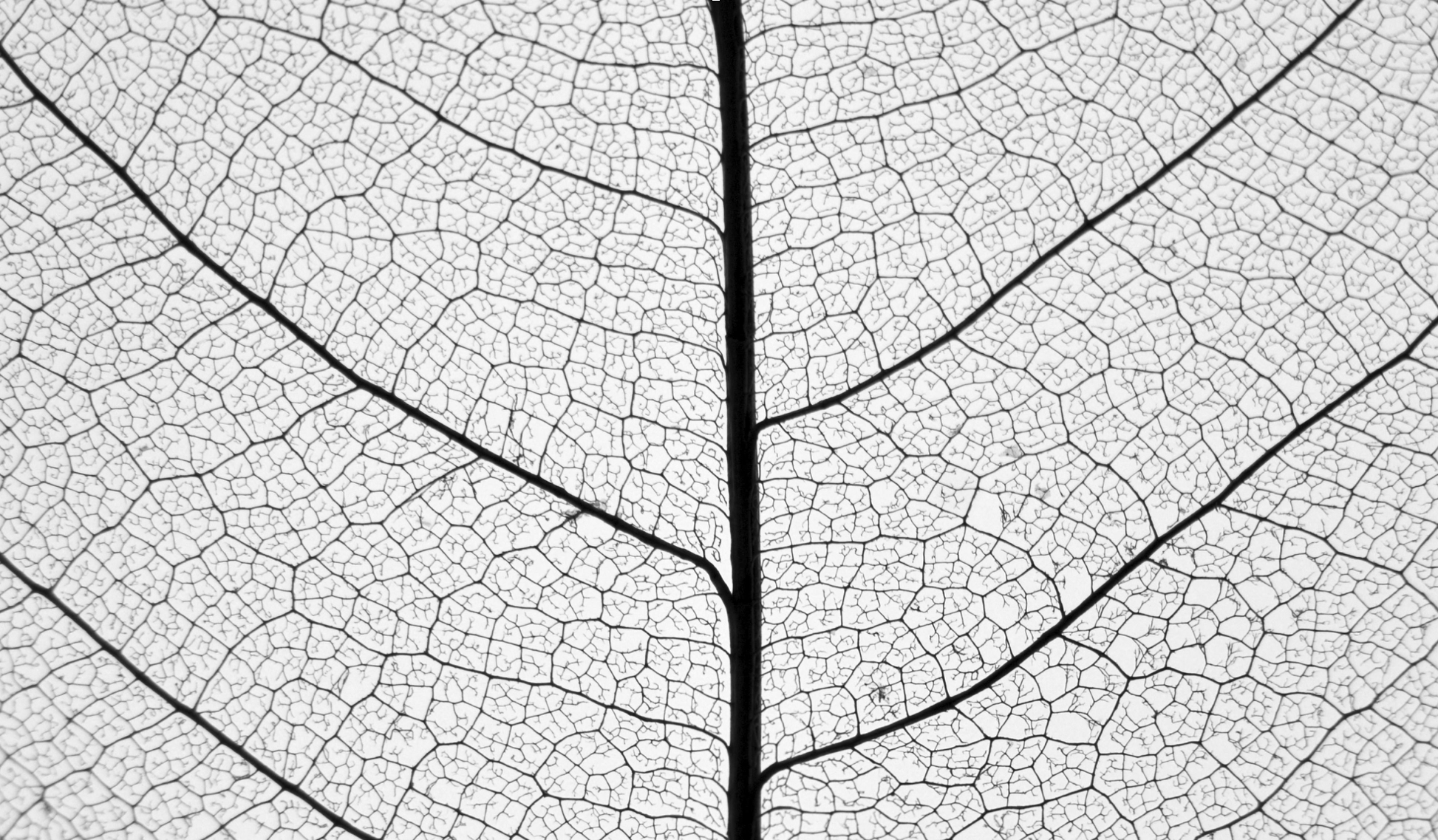
Panarchistic Architecture :: Chapter #4 [4.1]
Citation: Sterry, M. L., (2018) Panarchistic Architecture: Building Wildland-Urban Interface Resilience to Wildfire through Design Thinking, Practice and Building Codes Modelled on Ecological Systems Theory. PhD Thesis, Advanced Virtual and Technological Architecture Research [AVATAR] group, University of Greenwich, London.
4.1.3 Igne natura renovatur integra [through fire, nature is reborn][31]
“Fire is an expression of life on Earth and an index of life’s history.” Glikson and Groves, 2016.
Fusain deposits indelibly mark wildfires within the paleolandscape, and though much fragmented, nonetheless collectively constitute a record that is sufficiently rich as to provide a general understanding of the coevolution of fire and biota. For example, fossil data reveals that during the dry seasons of the Devonian [395 mya], progymnosperm forests persisted in conditions not unlike those of some Northern Hemisphere coniferous forests today (Cressler, 2001; Scheckler, 2001; Algeo et al, 2001; Pausas and Keeley, 2009). Then, as now, some forests experienced frequent, but relatively low intensity surface fires [32], which burned through dry shrubs and plant litter in the understory (Rimmer et al, 2015). Conversely, during the Carboniferous (345 mya), wetland forests dominated by the now extinct tree species Lepidodendron [33], together with relatively diverse flora including tree ferns [Fig. 14], coexisted with much longer fire intervals of between 105 and 1585 years (Falcon- Lang, 2000; Pausas and Keeley, 2009). However, in contrast to the surface fires of the progymnosperm forests of some 50 million years earlier, the Lepidodendron forests of the Carboniferous were subject to intense fires that would have engulfed large swathes of trees from ground to crown. As with the low intensity fires of the former, the high intensity fires of the latter evidence a symbiosis of biota and fire that, despite many millions of years of evolution, remains to the present day.
Whereas, historically, paleoecologists believed that plant evolution was tied only to climatological and alluvial shifts, a now sizeable body of data suggests that fire plays a pivotal role (He and Lamont, 2017; Pausas and Keeley, 2009). The intensity, frequency and behaviour of wildfires in and across landscapes vary greatly. ‘Fire regime’ is a term used to describe the relationship between a given ecosystem and variables including temporal nature [seasonality, frequency, and speed of spread], fuel type [ground, surface, and crown], spatial pattern [size and composition], and the scale and the nature of impact of the fire (Platt et al, 2015; Bowman et al, 2009; Heyerdahl, 1997; Agee, 1993, 1998; Heinselman, 1981). The primary variables used to classify fire regimes are fire frequency and severity (Heinselman 1973, 1978, 1981; Morgan et al, 1996, 2001; Hardy et al, 1998a, 1998b, 2000; Brown and Smith, 2000). More generally, an ecosystem’s affiliated fire regime is defined by the estimated frequency the data suggests fire usually returns to a given site (Jensen and McPherson, 2008), which can be expressed as its ‘mean fire-return interval’ [MFRI] (LANDFIRE, n.d). MFRI is not the only method employed to express the temporal- spatial relationship between fire and landscape. ‘Fire rotation period’ (Heinselman, 1973) is another commonly used term, as is ‘fire cycle’. But, whereas MFRI expresses spatial patterns within the landscape, fire rotation period and fire cycle represent the duration it would take for an equivocally sized area to burn (Morgan et al, 2001; Heinselman, 1973; Agee, 1993). For the purposes of this thesis the MFRI is the more relevant of the methods, because, as will become apparent in the case studies, fire spreads not uniformly within and across the landscape, but instead forms distinct broad-scale spatial patterns comprised burned and unburned patches of biota. The term ‘mosaic’ is used to describe this heterogeneous feature born of the behaviour of wildfires in landscapes. (Malamud et al, 2004; Turner and Romme, 1994).
“A fire is to a regime as a storm is to a climate” (Pyne, 2012, p.17), and an ecosystem is as tightly coupled to its fire regime, as it is to seasonal weather patterns. In turn, the many species of which an ecosystem is comprised are not adapted to fire in a generic sense, but, as with other environmental factors, including precipitation, humidity, solar and electromagnetic radiation, are adapted to one of several fire regimes, and to their affiliated mean fire return intervals (Swetnam and Baisin, 1996, Swetnam, 1993), suggesting that fire plays an integral role in selection for evolutionary fitness (He and Lamont, 2017; Hunter, 1990). The spatiotemporal dimensions of seasonality are governed by the configuration of continents, oceans, and atmosphere. Global seasonal variability in precipitation has been evident since the Carboniferous, becoming a primary determinant of fire regimes in the Paleogene, 25 mya (He and Lamont). Regional precipitation patterns dictate the possible sum and the general state of the biomass of an ecosystem. In the western United States this is evidenced by the impact of the jet stream, and more specifically, by the positioning of its northern and southern branches, each of which manifest very different weather conditions (Dettinger et al, 1998). The configuration of the wet/dry periods the jet stream [Fig. 15] creates is indicative of when, where and why wildfires will likely occur (Weisberg and Swanson, 2003; Grissino-Mayer and Swetnam, 2000; Morgan et al, 2001; Heyerdahl, 1997; Turner et al, 1994). Whereupon precipitation and/or humidity exceeds a certain level fire extent will be zero (Schroeder and Buck, 1970), because fire cannot burn whereupon fuel moisture surmounts the moisture of extinction [34], which averages somewhere between 12 – 40% of dry weight (Heyerdahl, 1997; Albini, 1976). Wind speed and direction plays a pivotal role in fire behaviour. High and low pressure systems are essentially a balancing act that develops due to temperature differentials in the atmosphere. High- pressure systems [anticyclones] create higher daytime temperatures, but lower wind speeds, and generally more settled conditions, and low-pressure systems [cyclones] vice versa. In both instances, wind speeds reflect the pressure gradient and the centrifugal forces [35] within the system. In the Northern hemisphere high-pressure systems flow clockwise, low-pressure systems flow counter-clockwise, and both their winds flow roughly parallel to the isobars (Met Office, 2013). Historically, the jet stream’s behaviour has manifested weather patterns that were particularly conducive to fires in the spring in the southwestern United States (Jensen and McPherson, 2008; Agee, 1998; Swetnam and Betancourt, 1990), and in mid-to-late summer in the Pacific Northwest (Agee, 1998; Wright, 1996). Regionally, cold fronts [36] are preceded by southeasterly to southwesterly winds, and are followed by westerly to northwesterly winds that bring cooler air flowing at a rate of 10-20 mph (Schneider and Breedlove, n.d). Whereupon atmospheric convection and moisture levels are sufficiently high a thunderstorm will ensue, and with it the sparks that may ignite the biomass below. In the event that a wildfire does start, its duration and direction may be influenced by Foehn winds, which known by local names, such as the Santa Anna and Mono in California, result from stable, high pressure air rising across and down the lee slopes of mountain ranges (Ibidem). More generally, unstable air manifests hotter wildfires that burn with greater intensity, and that are more likely to create blow-ups, which are sudden increases in both the rate of spread and intensity of the fire. In contract, stable air, and in particular the thermal belt that often rests along the mid-portion of mountainous slopes, can provide just the right combination of temperature invariance and low humidity to enable wildfires to actively burn through the night (Ibidem) [Fig. 16].
Solar radiation is a further systemic variable that is highly indicative of both wildfire probability and behaviour upon ignition. The spatiotemporal distribution of the Sun’s radiant energy is highly varied and dependent upon factors including solar irradiance [37] [i.e. the Sun’s rotation] (NASA, 2015); the gaseous and particulate composition of the atmosphere [i.e. aerosol levels] (NASA, 2017; Winckler, 2010), weather conditions [i.e. cloud cover] (Matusuzko, 2011), and landscape composition and type. Different species absorb solar radiation at different rates and in the case of plants the sum thereof is proportionate to the rate at which a species converts the radiation through the process of photosynthesis. In turn, ecosystems, and the biotic assemblages therein, greatly vary in their affiliated radiation absorbance/reflectance levels, as in turn do the microclimates that result therefrom (Chen et al, 1999). Biomass in direct sunlight can be as much as 10°C warmer than that which is in dense shade, which results in the former being easier to ignite than the latter (Schneider and Breedlove, n.d). In and of itself, at most, solar radiation can heat a surface to just over 71°C, which in the case of woody fuels is just over half of the minimum [204 - 371°C] required to create the combustion thereof (Ibid). Furthermore, studies evidence that, in some plant species, transpiration [38] is coupled with air temperature, wherein the higher the latter the greater the extent of the former (Crawford et al, 2012). Consequently, solar radiation simultaneously impacts upon surface air temperature, fuel moisture, and relative humidity [39], which collectively shape the combustibility of an environment. Thus, the “rhythms” of fire regimes (Pyne, 2012, p.25), and in more ways than one, a wildfire’s front [40] “links the atmosphere, biosphere, and hydrosphere via the release of heat, gases [notably water vapour], and matter” (Bowman et al, 2009, p.484), together with the pedosphere [41], therein might be conceived as ‘the point of convergence’ [42] of the Earth’s systems [Fig. 17].
>Continue to Chapter 4.1.4 here.
Footnotes
[31] Alchemists’ aphorism. No date or author known.
[32] Surface fires burn through ground-level vegetation and plant litter, including grasses, herbs, mosses, lichens, smaller shrubs, and saplings.
[33] Antecedents of modern-day mosses and quillworts, Lepidodendron selaginoides were tree-like vascular plants that flourished during the Carboniferous, persisting from 383.7 to 205.6 mya. Growing up to 30m high, the species was crowned by bifurcating branches baring large cone-like structures (Allaby, 2012; Toth, 2009).
[34] Moisture of extinction is parameter used in fire behaviour fuel models to predict spread rate. The latter increases the smaller the moisture-damping coefficient, which is calculated based on the difference between actual moisture content and the moisture of extinction (Rothermel, 1972; Scott, 2007).
[35] Born of Newton’s Second Law of Motion, the inertial Centrifugal Force references an apparent force that, directed away from the axis of rotation, acts upon objects within a rotating frame of reference (Wikipedia, 2017).
[36] Cold Front refers to a thermodynamical atmospheric process within which cold air forces warm air upwards, as it advances forwards (MET, 2016).
[37] Solar irradiance refers to the means by which the Sun’s radiant energy is measured and reported (NASA, 2008).
[38] Transpiration is the mechanism by which plants fulfil functions including homeostasis. Ascending from root to upper parts including the stomata [pores on leaf surfaces], 97-99.5% of the water consumed by plants thereon evaporates (Sinha, 2013), carrying heat energy away in the process.
[39] Relative Humidity is a function of moisture content and temperature, which expressed as a percentage represents the sum of moisture present relative to the point of saturation possible at air temperature. Therein, as a dimensionless ratio the function is ‘relative’ (NOAA, 2009)
[40] The wildfire front is defined as “the portion sustaining continuous flaming combustion, where unburned material meets active flames, or the smouldering transition between unburned and burned material” (Knowling, 2016).
[41] The pedosphere is the outermost of Earth’s layers, and comprised of soil, formed by “centuries old- effects’ of solar radiation, atmospheric moisture, vegetation, animals, and microorganisms on surface layers of rocks” (Dobrovolsky, n.d).
[42] “A sequence is “converging” if its terms approach a specific value as we progress through them to infinity” (Khan Academy, n.d). ‘The point of convergence’ is the finite limit within a sequence.
The thesis is also available in PDF format, downloadable in several parts on Academia and Researchgate.
Note that figures have been removed from the digital version hosted on this site, but are included in the PDFs available at the links above.
Citation: Sterry, M. L., (2018) Panarchistic Architecture: Building Wildland-Urban Interface Resilience to Wildfire through Design Thinking, Practice and Building Codes Modelled on Ecological Systems Theory. PhD Thesis, Advanced Virtual and Technological Architecture Research [AVATAR] group, University of Greenwich, London.