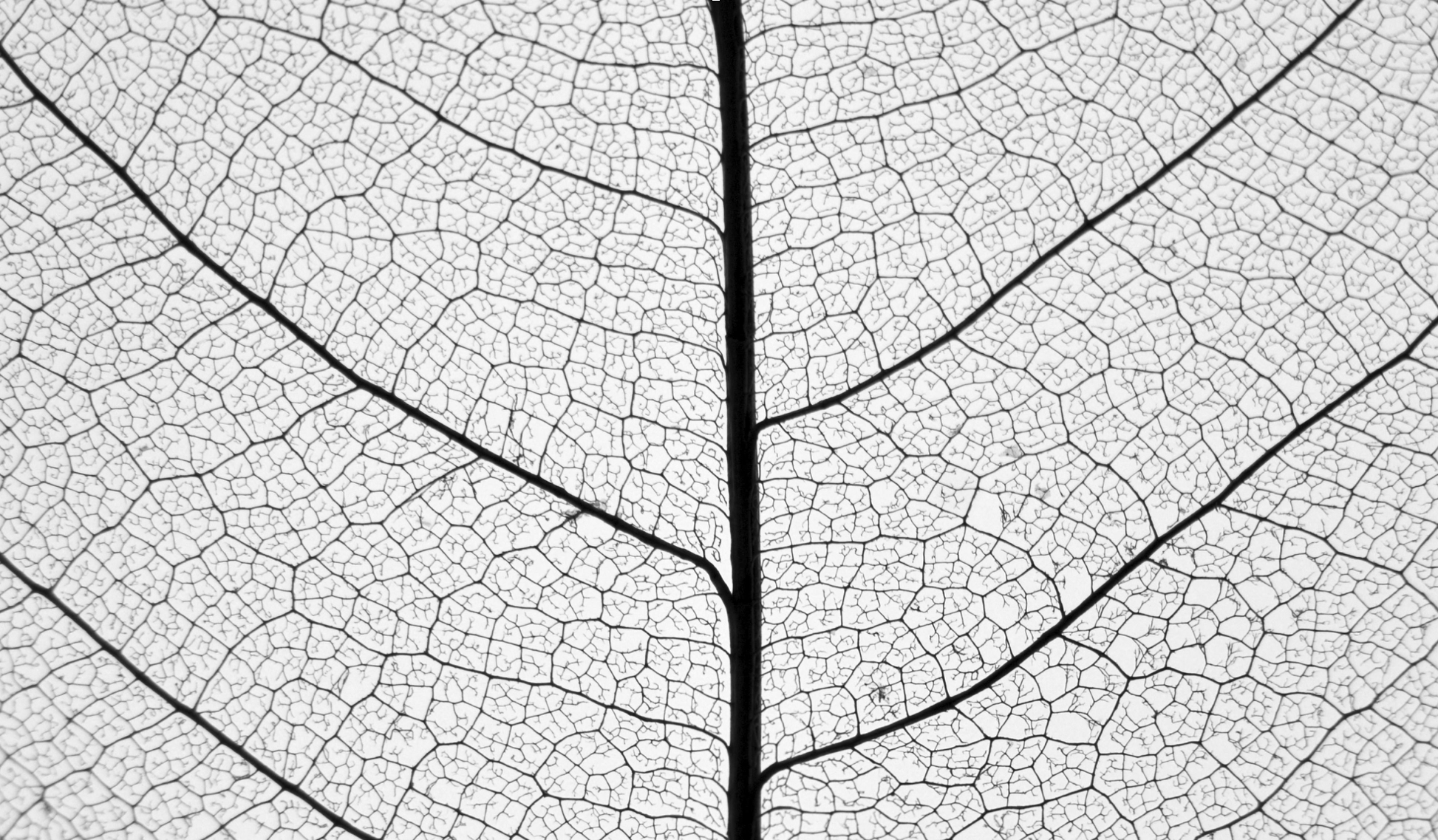
Panarchistic Architecture :: Chapter #4 [4.1]
Citation: Sterry, M. L., (2018) Panarchistic Architecture: Building Wildland-Urban Interface Resilience to Wildfire through Design Thinking, Practice and Building Codes Modelled on Ecological Systems Theory. PhD Thesis, Advanced Virtual and Technological Architecture Research [AVATAR] group, University of Greenwich, London.
4.1.4 Fire as Autopoietic Process: Feedback and Fluctuation in Fire Frameworks
“Our models attribute all morphogenesis to a conflict, to a battle between two or several attractions” Thom, 1972.
Whereupon viewing fire regimes through the lens of complexity, collectively, the distinct variants become the schemata, that being the term applied when describing the “patterns of behaviour and conceptual frameworks” within a complex adaptive system of which immediate behavioural changes are driven by feedback (Levin, 1999). Multiple studies have explored biomes as non-equilibrium systems (Archibald et al, 2013; Agee, 1998; Turner and Romme, 1994; Baker, 1989), some observing that within landscapes “temporal scale-free behaviour is evident in power spectra of fluctuations that obey power laws” (Bolliger et al, 2003, p.541), and this extends not merely to fire regimes (Green et al, 1990; Malamud et al, 1998; Song et al, 2001) but to evolutionary ecology (Bak and Sneppen, 1993; Sole et 1999), and, within the abiotic realm, to earthquakes (Gutenberg and Richter, 1954) and avalanches in sand piles (Bak et al, 1987, 1994, 1996), amongst other natural phenomena. Power-laws are evidenced in several studies of historical data sets of the spatiotemporal patterns of fire-prone landscapes (Malamud et al, 2005; Mortiz et al 2004); indicating common mechanisms are at play within these ecosystems. For example, upon analysis of a high-resolution data set of 88,916 wildfires in the conterminous United States in the period 1970-2000, a team led by Bruce Malamud of the Environmental Monitoring and Modelling Research Group at King’s College London found “robust” frequency- area power-law behaviour in 18 ecologically distinct regions (Malamud et al, 2005, p.4694). Their study also identified that “the ratio of the number of large to small wildfires decreases from east to west” (Ibidem, p.4697). Previous studies have suggested that the degree of connectivity between the biotic and abiotic units [i.e. density and configuration of biomass] is a primary factor in defining spatiotemporal patterns, with pre-existing conditions influencing the legacies left by fire disturbance (Bolliger et al, 2003). However, while correlating with the spatiotemporal pattern as would be expected given the regional climatic variations, Malamud et al acknowledged that, both spatially and temporally, so many are the variables within the ecoregions studied that multiple feedback mechanisms are likely impacting on wildfire behaviour.
Feedback mechanisms within the schemata of fire regimes vary in both speed and strength. The term slow variable describes that which, relative to the fast variable, usually shapes the system parameters considerably more slowly (Walker et al, 2012; Gunderson et al, 2002, 2010; Ludwig et al, 1978). During the Devonian and the Carboniferous, the climate and atmospheric composition were slow variables at play in fire regime schemata, whereas the weather was a fast variable. For example, during the Devonian Earth’s biomass expanded 800-fold (Borzenkova and Turchinovich, 2009) which set in motion a steep decline in atmospheric CO2 (Vecoli et al, 2010), coupled with a sharp rise in atmospheric O2. Consequently, the atmospheric conditions were comparatively favourable for fire to those of the early Triassic [252 mya], the latter a period in which atmospheric O2 fell as low as 12%. However, while the fossil record evidences a general correlation between atmospheric O2 levels and wildfire activity, fusain deposits from the Triassic period speak to not one, but several variables at play within the wildfire schemata [Fig. 18] (Abdullah et al, 2012; Uhl et al, 2008; Berner et al. 2003). Whereupon contemplating what other variables may have contributed to the sharp decline in wildfire in the period, the Permian-Triassic extinction [P-Tr] event [c. 252 mya] may provide some answers. The P-Tr, which is colloquially referred to as the ‘Great Dying’, resulted in a dramatic drop in both species populations, and the distribution thereof (Shen et al, 2011; Benton, 2005). While the fossil records suggest that flora weathered the P-Tr relatively well, with some 50% of plant families surviving (McElwain and Punyasena, 2007), given the immensity of the faunal species that went extinct, we might reasonably assume that the P-Tr significantly impacted upon the functioning of early Triassic forest systems. For example, studies of diverse living ecosystems strongly support the statement that faunal assemblages play a central role in shaping plant communities, including both population and distribution of species [43] (Ripple, 2014; Cromsigt and te Beest, 2014; Haynes, 2012; Wallace, 2004; Levin, 1999; Folke, Holling and Perrings, 1996).
Applying the logic of Occam’s Razor [44], a reduction in faunal diversity may equate to a reduction in floral diversity, therein the available fuel source [hydrocarbons], which in turn led to a decline in the number, and possibly the size, of wildfires. Fossil records evidence that as species populations declined at the end of the P-Tr, soil erosion was rampant (Sephton et al, 2005), as was fungal virulence (Visscher et al, 2011), both of which are linked to deforestation. The former, soil erosion, is suggestive of fires so intense as to burn sufficient vegetation as could cause soil to lose its structural integrity [i.e. loss of root systems], therein easily displaced by heavy precipitation. The latter, fungal virulence, suggests that forests were comprised of sizeable quantities of dead and decaying trees [i.e. tinder-dry fuel] most likely incurred by the rapid shift from a wet to arid climate, for which some species were not adapted. Therein, the above hypothesis works within the wider framework of our evolving understanding of the relationship between biota and fire.
Historically, landscape topography is a generally slow variable within fire regimes. Studies evidence that the location, size, elevation, aspect [exposure to solar radiation], and form of mountains, slopes, and water bodies have a bearing on the spatiotemporal dimensions of wildfires (Wallace et al, 2004; Heyerdahl, 1997), impacting on fire’s rate of spread, direction, and intensity (Schneider and Breedlove, n.d). Whereas landscape gradient influences the speed, and in some instances, the direction of a wildfire front, lakes and wide-rivers act as boundaries that can either slow or stop a wildfire front from progressing in a particular direction. A wildfire’s rate of spread increases on uphill gradients, which is due to the upward motion of the flame front in combination with the processes of convective and radiant heat transfer preheating the fuels above (Ibidem), thus priming them for combustion. The rate of spread is relative to the gradient: “the steeper the slope, the faster the fire burns” (Ibidem, p.8). A wildfire’s rate of spread will generally slow on a downhill gradient, however, falling and rolling burning debris will often ignite one, if not several, secondary fires below the main fire (Ibidem). Landscape configurations known to compound a fire’s rate of spread and intensity include: Box Canyons, that in similar fashion to a tower block juxtapose steep walls against a level base, of which the thermodynamical consequence is ‘the chimney effect’ [strong updrafts], therein a rapid rate of spread, this being a behaviour also affiliated to other topographic features with steep walls, including both narrow and wide canyons (Ibidem).
While topographic features are, ultimately, impermanent within the landscape, most often they remain in situ for lengthy periods of time, thus usually constitute a slow - sometimes extremely so - variable. For example, tectonic reorganisation within the landscape has been attributed as one of the causations of a wide-scale reduction in the number of coal-forming forests during the Permian [45] [299-251 mya], which is cited as a possible driver in the decline of wildfires in the period (Uhl et al, 2008). However, whereupon reducing the temporal scale from many millions of years down to decades, we find topographic shifts reducing from the global to the local in scale. In living forests causations for topographic shifts include geological failures, such as landslides and earthquakes, volcanic eruptions and the secondary impacts thereof, such as lahars and debris flows, and anthropogenic activities, including mining and road building. Thus, in some instances, landscape topography can become a fast, not a slow variable in the fire regime schemata, and particularly when a natural hazard has recently occurred.
Comparative to the weather, a fire regime’s biota is a slow variable, in so far as its mass [i.e. fuel load] needs to have reached a critical threshold to sustain a fire within a landscape, this being a process that at the minimum takes several months or more (Heyerdahl, 1997). However, whereupon one changes the temporal scale from months, years, decades, or centuries to millennia, the biota becomes a comparatively fast variable compared to the climate and atmospheric composition. Furthermore, as will become evident in the case studies to follow, feedback between the variables of fire regimes, and in particular between wildfire and biota, creates not only spatial complexity, but also temporal memory effects (Malamud, 2005). A great many species indigenous to fire-prone regions exhibit lifecycles that are synchronous to fire regimes [Fig. 19], of which examples will be given below. Indeed, some species even exhibit mechanisms that propagate fire by means of creating conditions that are conducive to their successful reproduction, thus shape pre and post-fire conditions in more or less equal measure. The interplay between the biotic and the abiotic is sufficiently intimate as to constitute a synecological unit akin to the many that have been observed both in and between floral and faunal species groups, such as angiosperms [flowering plants] and pollinators. Therein, one might plausibly argue that environmental management, and no less so than in the case of fire regimes, is not the exclusive domain of humanity; biota exhibit many, varied, and often subtle ways of influencing the environment, be it through reproductive processes, or other means, including, but not limited to allelopathy [46], epiphytism [47], and nitrogen [48] and carbon fixation [49]. However, whereas some, but not all interspecies relationships are to the benefit of one, but not the other [i.e. parasitism], the relationship between fire-adapted species and their affiliated fire regimes are not merely symbiotic, but mutualistic [50]; an interdependent symbiosis. Thus, one might argue that fire ecology extends the concept of autopoiesis [51] (Maturana and Varela, 1973) beyond the realm of the living, to encompass both the life and the physical sciences: the natural sciences. But, the spectrum of the parameters of the autopoietic processes within the synecological unit that the biotic and abiotic components of fire regimes collectively comprise are yet to be firmly established (Bolliger et al, 2003; Malamud, 2005).
>Continue to Chapter 4.1.5 here.
Footnotes
[43] Faunal assemblages shape floral communities in wide-ranging direct and indirect ways, examples include; the grazing habits of megaherbivores influencing both the composition and structure of savannah grasslands (Cromsigt and te Beest, 2014; Haynes, 2012), and large carnivores feeding behaviour affecting stream morphology, carbon storage, and disease dynamics (Ripple, 2014).
[44] Attributed to the 14th century Franciscan friar, theologian, and philosopher William of Ockham, ‘Occam’s Razor’ is a heuristic principle used in the development of theoretical models as a basis for methodological reductionism. Originally expressed in the statement "Pluralitas non est ponenda sine neccesitate" [Entities should not be multiplied unnecessarily], among competing hypotheses, it advocates that which has the fewest assumptions (Gauch, 2002).
[45] Several different dates are in use for the Permian. The period 299-251 mya is cited from International Commission on Stratigraphy’s International Chronostratigraphic Chart v2016/12.
[46] A form of biogenic toxicity, allelopathy involves “the chemical inhibition of one organism by another” (Lincoln et al, 1998).
[47] Epiphytes are organisms that grow on the surface of plants by means of support or anchorage, with examples including several species of vines and climbers, such as ivy and honeysuckle (Lincoln, 1998).
[48] Nitrogen fixation is the process by which atmospheric nitrogen or molecular dinitrogen is reduced and incorporated into nitrogenous compounds, including ammonia. The process can occur through both abiotic and biotic processes, of which the former are photochemical reactions in the atmosphere. The latter occurs by the action of nitrogen-fixing bacteria, including Rhizobium and Bradyrhizobium [which form the root nodules of leguminous plants], and cyanobacterium [occurring in some lichen species] (Allaby, 2012). Nitrogen is essential for the production of the complex molecules with which life is built, including nucleotides (in DNA and RNA), amino acids (in proteins), and nicotinamide adenine dinucleotide (in metabolism); therein its fixation forms the foundation of Earth’s ecosystems.
[49] Carbon Fixation is “the conversion of inorganic carbon into energy-rich organic carbon by photosynthesis (Lincoln, 1998).
[50] Mutualism is an interaction, and frequently interdependence, between species populations wherein the parties benefit mutually (Lincoln, 1998).
[51] A concept introduced in 1972 by biologists Maturana and Varela, ‘autopoiesis’ refers to a system’s capacity to autonomously repair and reproduce itself.
The thesis is also available in PDF format, downloadable in several parts on Academia and Researchgate.
Note that figures have been removed from the digital version hosted on this site, but are included in the PDFs available at the links above.
Citation: Sterry, M. L., (2018) Panarchistic Architecture: Building Wildland-Urban Interface Resilience to Wildfire through Design Thinking, Practice and Building Codes Modelled on Ecological Systems Theory. PhD Thesis, Advanced Virtual and Technological Architecture Research [AVATAR] group, University of Greenwich, London.